Review Essay: Other Minds
If you venture too deep into other kinds of minds you never know what you will finds.
“When you work with fish, they have no idea they are in a tank, somewhere unnatural. With octopuses it is totally different. They know that they are inside this special place, and you are outside it. All their behaviours are affected by their awareness of captivity.”
Stefan Linquist
Octopuses track you. They will watch you as you approach them, and monitor what you’re doing. When they’re in aquariums and tanks, they often try to escape, and to do so, they wait until you’re are not looking before they make their move.
One of the first encounters with an octopus often involves touch. This is a passage from Other Minds, by Peter Godfrey-Smith (henceforth, PGS):
This octopus is in a den. Shells are strewn in front, arranged with some pieces of old glass. You stop in front its house and the two of you look at each other. This one is small, about the size of a tennis ball. You reach forward a hand and stretch out one finger, and one octopus arm slowly uncoils and comes out to touch you. The suckers grab your skin, and the hold is disconcertingly tight. Having attached the suckers, it tugs your finger, pulling you gently in. The arm is packed with sensors, hundreds of them in each of the dozens of suckers. It’s tasting your finger as it draws it in. The arm itself is alive with neurons, a nest of nervous activity. Behind the arm, large round eyes watch you the whole time.
Octopuses often try to drag humans into their dens. This isn’t necessarily because the octopus is trying to eat people, although those legends about the kraken did come from somewhere - instead it seems to be because they like to play with things.
For instance, when PGS tried to place stakes in the ground underwater in order to monitor an area which octopuses were known to frequent (he calls it Octopolis, charmingly), he would often come back the next day to find that the stakes had been moved, and tossed on a scallop heap.
Basically, octopuses like to dick about.
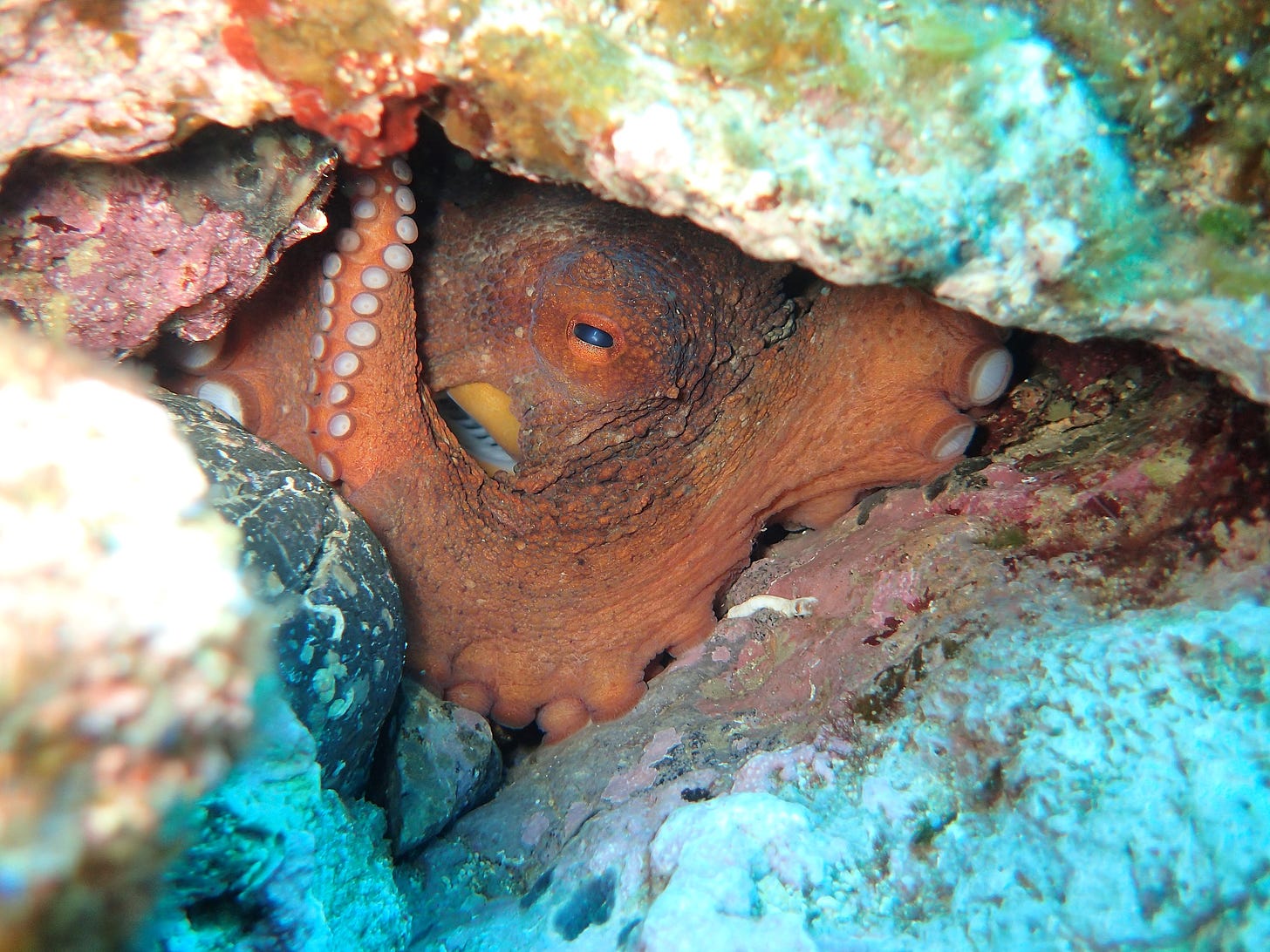
One story comes from an aquarium in Germany, where an octopus named Otto had been causing powercuts by blasting streams of water at a light above his tank. Otto would also pass the time by juggling hermit crabs, throwing stones at the glass, and rearranging the contents of his tank in order to more properly align the feng shui with his own tastes, causing much distress for the tank’s other occupants.
Another story comes from a laboratory in Pennsylvania, where octopuses were fed on squid. Octopuses don’t like squid that much, generally, although it’s not hard to imagine that the taste palettes of octopuses vary drastically. Most of the octopuses were chowing down on their squid, apart from one, which waited until the researcher was watching it, and then, clearly making eye contact with the researcher, slowly placed the squid in the drain, which promptly ejected it from the tank.
Jon Ablett, the cephalopod curator at the Natural History Museum, whose job title must go down in the annals as ‘relevant to this post’, recalls a story about one lab, where “all the fish were going missing from their tank. The staff set up a little video camera and it turned out that one of the octopuses was getting out of its tank, going to the other tank, opening it, eating the fish, closing the lid, going back to its own tank and hiding the evidence”.
These sorts of stories abound. PGS says at one point that octopuses learn things, but slowly. This is maybe true in the lab, but deeply untrue outside of the lab. Binyamin Hochner, one of the doyens of octopus study, writes in a 2006 paper that:
The development of sophisticated motor, sensory, and cognitive capabilities, such as excellent vision (for review see Budelmann, 1996; Muntz et al., 1999; Williamson and Chrachri, 2004), highly efficient flexible arms (Yekutieli et al., 2002; Flash and Hochner, 2005), and the ability to learn rapidly (Wells, 1978; Mather, 1995; Hanlon and Messenger, 1996; Nixon and Young, 2003) allowed the dramatic changes in cephalopod behavior necessary for successful competition with the vertebrates (Packard, 1972; O’Dor and Webber, 1986). [Emphasis mine]
That Wells book, Octopus: Physiology and behavior of an advanced invertebrate, refers to them as “learning machines”. It is likely that perceived limitations in octopus learning come from the fact, to put it bluntly, that they don’t give a fuck about the task humans are prodding them to do.
Here’s what I mean: In one experiment on bees, scientists got bees to fly to a square or a triangle, and landing on the correct, arbitrary, shape would reward the bee with some food. The bees would just rapidly fly to each shape until they got food. Scientists assumed from this that bees couldn’t recognise shapes. Scientists assumed this for decades.
It turns out bees were doing what many humans would do - if a task is hard, and there’s no punishment for failure, just press buttons until you get the result you want. In another experiment, where bees were given food laced with quinine (which they hate) for landing on an incorrect shape, the bees tightened up their game immediately, and were much more accurate.
Something similar may be happening with octopuses. Beyond needing to eat to live, there’s no reason an octopus would be particularly desperate to jump through the hoops provided by human experimenters. They behave exactly how you might expect an animal with excess resources in captivity might behave.
They explore. They play. And they break things.
In the 1950s, a Harvard behavioural scientist called Peter Dews wanted to find out whether octopuses could learn to pull a lever to get food. He had three octopuses named Albert, Bertram and Charles, and writes of the results:
Whereas Albert and Bertram gently operated the lever while free-floating, Charles anchored several tentacles on the side of the tank and others around the lever and applied great force. The lever was bent a number of times, and on the 11th day was broken, leading to a premature termination of the experiment.
The light, suspended a little above the level of the water, was not the subject of much “attention” by Albert or Bertram; but Charles repeatedly encircled the lamp with tentacles and applied considerable force, tending to carry the light into the tank. This behaviour is obviously incompatible with lever-pulling behaviour.
Charles had a high tendency to direct jets of water out of the tank; specifically, they were in the direction of the experimenter. The animal spent much time with eyes above the surface of the water, directing a jet of water at any individual who approached the tank. This behaviour interfered materially with the smooth conduct of the experiments, and is, again, clearly incompatible with lever-pulling.
The behaviourists were an odd bunch, and this account is oddly phrased. Despite what Dews says, none of Charles’ additional non-lever-pulling-behaviours are actually incompatible with lever-pulling, unless the octopus is supposed to spend all day grindin’ lever reps at the lever gym and downing packets of shrimp protein powder to pick up lever babes. Behaviourists placed an undue emphasis on reward, and focusing only on what could be observed (i.e., negating the inner life). Note the placement of “attention” in inverted commas, as if Dews is sceptical that such a thing could exist.
Charles exhibited a similar response to many octopuses, who are not so interested in the task structure and more in the fact they are in an unnatural environment. In fact, Papini and Bitterman (1991) notes that in one experiment, octopuses often didn’t hit task-success thresholds of 80% because they were too busy deliberately choosing the wrong choice to see what happened.
VERY NERVOUS SYSTEMS
Briefly, the relevant parts of the taxonomic tree for cephalopods looks like this:
I refer to cuttlefish and octopuses pretty interchangeably throughout this post, but I should make it clear that each of these creatures are different from each other, and from other members of their own order - for instance, there are about ~300 species of octopus. There are a lot of similarities too, but just bear that in mind.
So, what are we actually trying to understand about cephalopod cognition? Is it self-aware? Can it recognise itself? Does it have a good memory? Can it recognise other members of its species? Can it recognise individuals which are not members of its species? How does it communicate? Is it social, and what do its social interactions look like? Can it use tools? What complex motor patterns does it use? Are there other unique behaviours that are interesting?
That isn’t an exhaustive list, but when I try to think about cognition in the minds of animals, including humans, I’m usually tossing these sorts of questions around in my head.
Only 3 of the 34 animal phyla have what Michael Trestman calls “complex active bodies”. These are chordates (anything with a spine, so me once I’ve had a few beers), arthropods (insects and crabs) and a small group of mollusks, the cephalopods. Complex active bodies are what you might expect - basically any creature that does complicated and interesting things - but Trestman gives specific criteria:
articulated and differentiated appendages
many degrees of freedom of controlled motion
distal senses (e.g. ‘true’ eyes)
anatomical capability for active, distal-sense-guided mobility (fins, legs, jet propulsion, etc.)
anatomical capability for active object manipulation (e.g. chelipeds, hands, tentacles, mouth-parts with fine-motor control)
The last common ancestor of cephalopods and chordates had neurons. It was a worm-like creature, with a simple nervous system. It may have had simple eyes.
Our nervous systems are relatively centralised. They may have developed from two discrete nervous systems, one of which provided sight and one of which provided internal communication, in the way that a nervous system does. These two systems may have then grown towards each other. I have to keep saying may in inverted commas, because it is not clear.
We don’t know much about what life looked like ~540 million years ago. For instance, we will probably never know when animals start to be able to see in colour, because cone cells, which let you see in colour, do not survive in the fossil record. Perhaps there is another way to work this out, but as far as I know, no-one has found it yet.
On the other hand, cephalopods have distributed nervous systems. Invertebrate neurons are collected into ganglia, small knots which run along the body and across it. This often looks like a ladder, and is sometimes imaginatively called a “ladder-like” nervous system. Here’s a flatworm’s nervous system, a simple version of this type of system:
Octopuses have twice as many neurons in their arms as they do in their central brain. Note that octopuses have arms, not tentacles, because arms have suckers all the way along, like a crypto scam, whereas tentacles have suckers only at the end, like a different type of crypto scam.1
The arms have their own sense of taste, and they can sense chemicals. Each sucker has around 10,000 neurons. Even an arm that has been surgically removed can still perform actions like grasping and reaching, and can probably feel pain.
When an octopus grabs something, there are two simultaneous waves of electrical activation - one coming from the tip of the arm, and the other from the base of the arm. These activations meet in the middle, forming a joint.
Here’s Carls-Diamante breaking down the octopus nervous system:
What is significant and fascinating is the extensive functional autonomy of the three components of its nervous system: the central brain, the optic lobes, and the peripheral arm nervous system. Even more surprising is how the neurons are distributed among these parts: the central brain contains roughly 45 million, the paired optic lobes have 120 million between them, and the arm nervous system has 350 million—two thirds of the octopus’s total neurons. The high concentration of neurons in the octopuses’ arms is consistent with the extent of information processing that takes place in the arm nervous system. Each arm receives massive amounts of sensorimotor information from millions of receptors in the suckers, skin and muscles.
The nervous centers of each arm are responsible not only for processing sensory information, but also for controlling reflexes and issuing motor commands to their respective appendages. This information is processed locally by nervous centers within each arm, and consolidated in the interbrachial commissure, a ring of fibers that interconnects the arms.
I’ve mentioned corollary discharge (CD) in the past, where animals generate internal copies of signals in order to deal with the problem of reafference. The problem of reafference relates to correctly updating your map of the world in response to your own actions. Simply put, if you move, you change the inputs you see, so you have to then factor that in when building a picture of the world.
All nervous systems partake in CD. For instance, everyone’s favourite nematode, C. Elegans, only has 302 neurons. But it has a CD mechanism, and it’s a very exciting one: whenever C. Elegans moves forward, it activates a CD mechanism, which prevents a reflex arc which would counteract that move and send C. Elegans backwards. This, thrillingly, allows C. Elegans to go forward.
The crayfish responds to danger in its environment by tail-flipping to safety - don’t try this at home, especially if you’re driving. It uses GIANT COMMAND NEURONS (which I’ve put in caps because the term is exciting, rather than significant), which issue CD commands for when it might not want to tail-flip, or when it needs very desperately to tail-flip.
Finches use CD in order to learn songs. Finches learn songs in three stages; they listen to other finches sing songs in their environment, then they mimic those to start producing vocalisations, then they hone these vocalisations into their own beautiful song. If they don’t hear the other finches, they produce songs, but the songs are bad. How do you measure if finch songs are bad? Finches use their music to attract mates, and if the songs don’t attract mates, they’re bad songs. Music criticism is simpler in the world of finches.
During that second stage, where juvenile finches are comparing their own vocalisations to adult finches, they don’t listen to their own auditory feedback. They instead listen to a prediction of what their song sounds like, provided by CD mechanisms, which operates in between the finches’ memory of the model song and their own vocalisations. And how do we know that this is happening? As the authors of this paper put it: “The use of efference copy as a substitute for the sensory signal explains the ability of adult birds to produce normal song immediately after deafening”. If finches were relying on auditory feedback, deafening them would ruin their own songs.
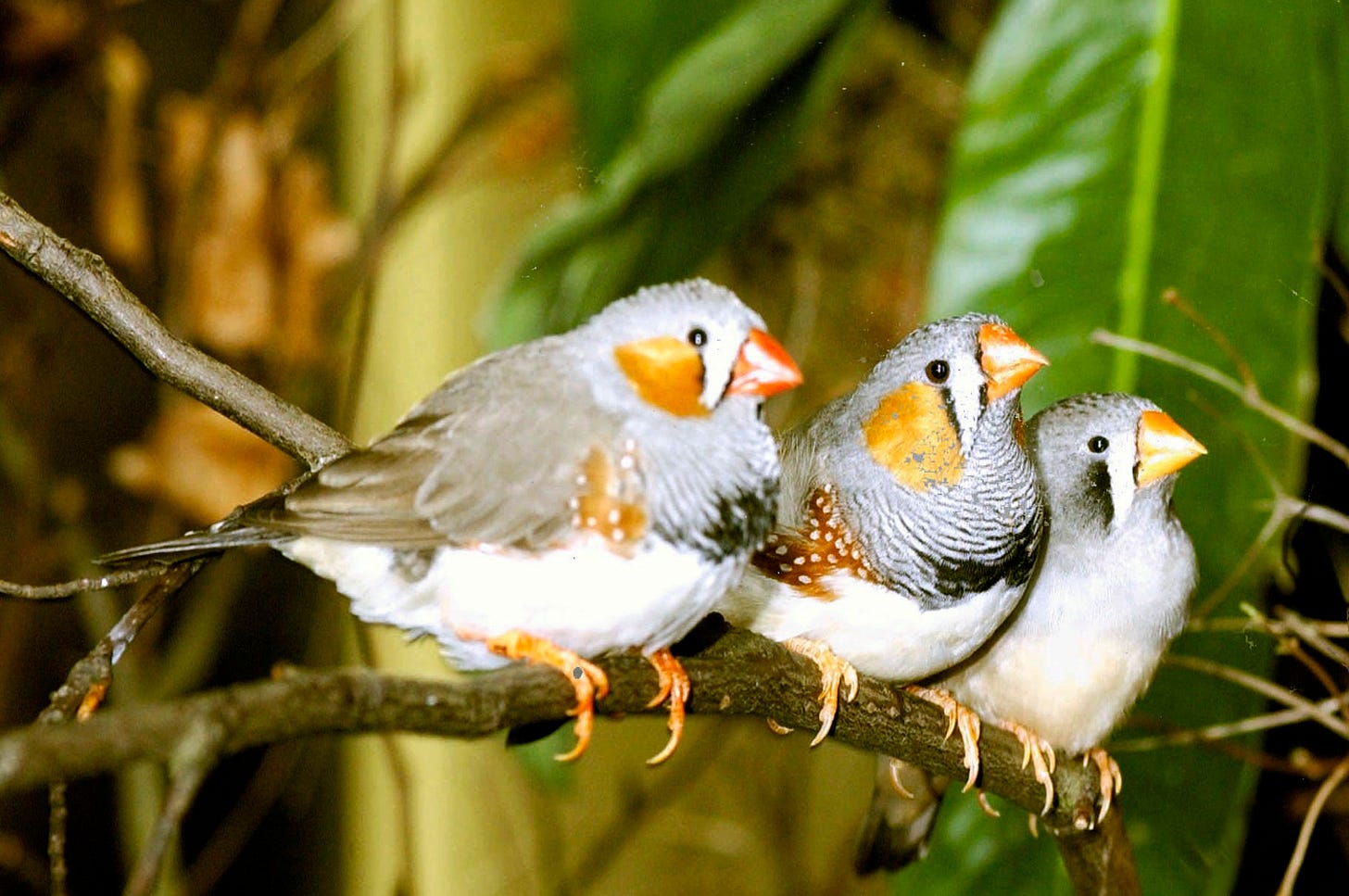
At higher levels of the neural hierarchy, primates use CD in order to allow eye movements to work. When we look at the world, the eyes jump around to different places of interest. These jumps are known as saccades. If we saw what our eyes were actually seeing, it would be a terrifying image - constant jumping, fragmented images of the world that would seem disconnected and incoherent.
The brain uses a CD system in order to suppress information that comes from these saccadic jumps - but which factors in movement in the environment, so that we are not entirely exposed to a random drop-bear attack during these saccades.
Why did I just drone on about corollary discharge for six paragraphs? Because these CD commands, unless they are very simple, usually go “up” the neural hierarchy, towards decision-making areas. They’re part of predictive networks that in humans, are thought to combine top-down and bottom-up sensory processing, but which may do similar things in other species.
So what happens in octopuses with corollary discharge? How do octopuses integrate all their electrical data, when all their neurons are scattered about their body?
Octopuses do have a brain that has some control over the rest of the octopus. But the arms are operating in tandem, and as I mentioned above, can operate without the brain at all. PGS suggests that cephalopod brains don’t have reafference copy of the actions of the octopus. Not having a copy of its actions must shape the octopus' experience of the world in a critical way.
Basically, the brain probably has no sense of where its arms are.
If you watch an octopus walk like this (source):
It certainly looks like the arms are being co-ordinated centrally to generate movement, but this is not likely. The brain is probably selecting which arms are getting used, and activating a program that says, “Let’s walk now”, and the arms in turn begin their patterns of fine-grained motor commands, specifically operating a pushing by elongation motor pattern.
Arm co-ordination usually requires some ability to utilise top-down control. Your (probably human) somatosensory cortex will have areas specifically dedicated to processing feedback from your hands, or your feet. Review papers show that the octopus brain doesn’t form somatotopic representations of the arms, and that it can’t determine where the arm is located in space. Proprioception is your sense of where your body is in space, and it is thought that this is linked to somatosensation. So how does proprioception work in the octopus? The answer is that it doesn’t. Octopus brains have no sense of where their arms are.
There are thin neural connections which run from the arm to the brain, but they are so thin that this suggests that considerable information processing has already occurred before the information gets anywhere near the brain.
There seems to be a set of ‘opt-in’ CD circuitry, where the arm can provide feedback to the brain, via the ganglia, in response to complexity in the environment.
Because while the arms seem to operate independently, they can also, with some pushing, become subjected to a centralised control. Octopuses will tend to damaged arms by pulling the wound close to their mouth, evaluating the damage and running other suckers along it.
In one study, scientists showed that octopuses could guide their arms through a maze towards a piece of food that they could only see visually - the arm had to leave the tank at one point, so scientists are pretty sure it was being guided, because octopus arms cannot chemically sense when they are not in the water.
It seems as if the arms and brain may choose, in response to any task that is more difficult than their usual fare, to collaborate.
Then, once a task like the magical food maze has been mastered, the arms return to a state of higher autonomy, and resume exploring the environment as the octopus moves.
PLAY, COLOURS AND DEATH
So that was a lot of words to say that basically, octopuses are strange. They’re often referred to as intelligent aliens, but the word alien feels like an understatement when it comes to thinking about octopus minds.
Octopuses can’t recognise themselves in the mirror test, where you put a dot on an animal, show them a mirror image of themselves, and see if they try to remove the dot. But they can differentiate between humans, because they fire jets of water specifically at experimenters they don’t like. Octopuses may have good other-vision and bad self-vision.
They have been known to play a version of marine tennis, with two octopuses blasting jets of water at a floating pill bottle across a tank. Some octopuses don’t do this. Some do. The ones that do follow a similar sequence to children interacting with new objects, where they move from “What is this object?” to “What can I do with this object?”, with the answer being tennis.
The basic problem with all octopus experimentation is that they have huge individual variation. Some are generalists when it comes to hunting, and use generalised tactics to hunt lots of species. Some are specialists, and use specialised tactics to hunt a small number of species. All of them vary in their response to experimental procedures, and consequently experimental procedures take a long time. If a particular tactic works they use it until it doesn’t anymore, and if it doesn’t work they try a bunch of different things until something does work.
PGS spends a lot of time discussing colour changing, which he sees, at least in part, as an expression of internal states. This seems plausible. Octopuses are likely to be colour blind, as they only have one type of photoreceptor, and colour perception usually takes two. And yet they do things like this (source):
Who is this for? Some of it is clearly for camouflage. When octopuses ditched their hard shells millions of years ago, it made sense to develop alternative defensive tactics, and they will use colour to blend into their environments, or to generate different deimatic displays to confuse predators. But PGS gives multiple accounts throughout Other Minds of octopuses or cuttlefish, sat still in the water, changing colours. They weren’t responding to him either, because they did exactly the same thing on camera, when he wasn’t nearby. He theorises:
I think that some cephalopods, especially cuttlefish, have an expressiveness that goes beyond anything with a biological function. Many patterns seem to be anything but camouflage, and are also produced when no obvious “receiver” of the signal is around. Some cuttlefish, and a few octopuses, go through an almost continual, kaleidoscopic process of colour change that appears disconnected from anything going on outside them, and appears instead to be an inadvertent expression of the electrochemical tumult inside them. Once the color-making machinery on the skin is wired to the electrical network of the brain, all sorts of colours and patterns might be produced that are simply side effects of what is going on within.
PGS also notes that cephalopods usually have brief life spans of around one year. This is relatively short. There is some variation, and in deeper waters the lifespan can increase, but all metabolic processes occur more slowly in colder environments like deeper water. So giant pacific octopuses can live for around 16 years, because they live in very deep water.
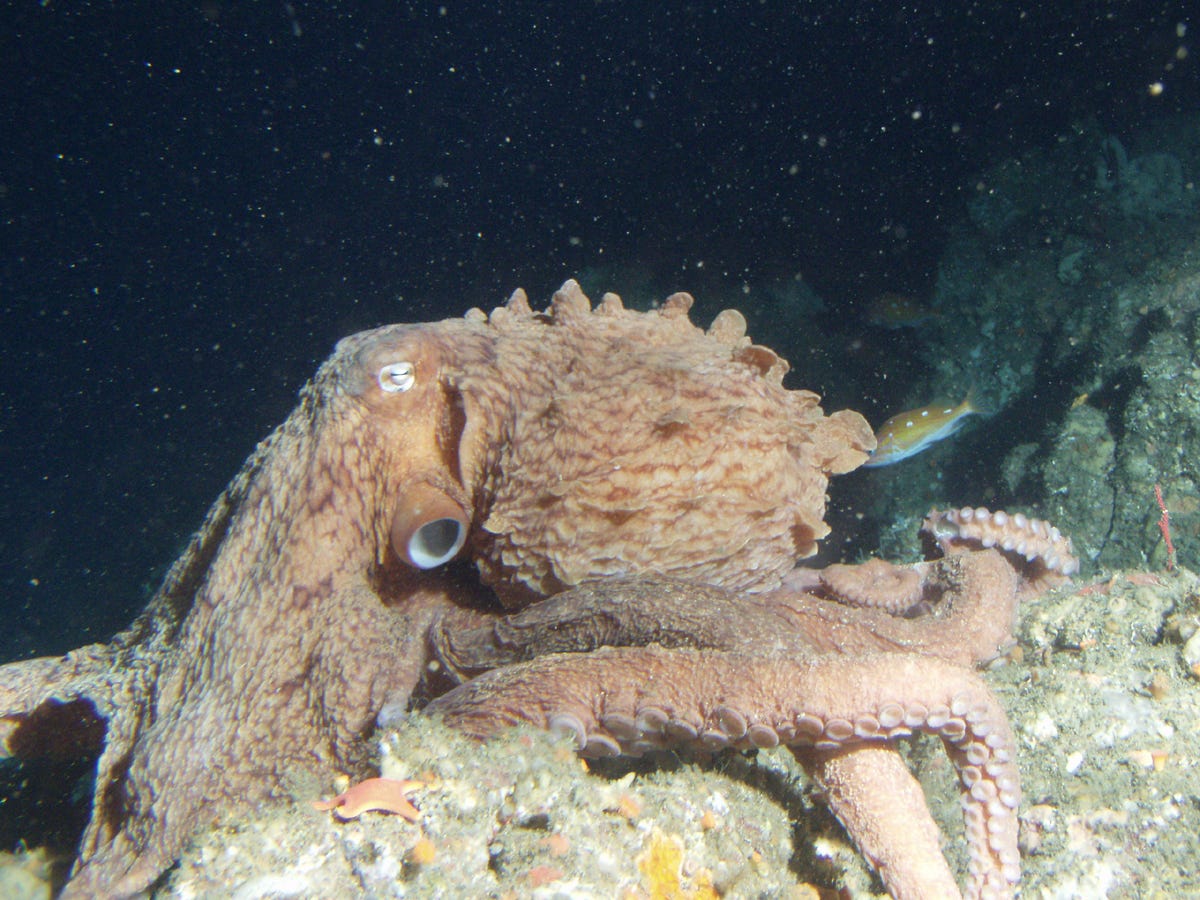
The reason for their short lifespan is probably because they are relatively defenseless. When they lost their shells, cephalopods gained the opportunity to develop large brains, but also massively increased the risk that they would be eaten by something. If you are an octopus, it makes evolutionary sense to become fertile quickly, have as many children as possible, and then fall apart. So this is what they do. This is known as semelparous reproduction.
Octopuses also live solitary lives, largely due to cannibalism. Eggs from the same mother are scattered widely throughout the open ocean, and consequently there is little opportunity to help kin. It is safe to assume, if you are an octopus, that everything is out to get you, including your fellow octopuses.
THE C-WORD
So what do we know about the inner lives of octopuses, and other cephalopods? Do they have conscious experiences? What is it like to be an octopus?
It’s never clear what consciousness is like for another species, or even for another human. Many of the fiercest debates between people are likely because they inherently see the world in fundamentally quite different ways, and struggle to reconcile themselves to this disparity.
One of the best parts of Other Minds was PGS’ contribution to this question of octopus consciousness. He doesn’t tackle it head on - there is no good way to hit consciousness head on - but he shows, in a gentle but persistent manner, how consciousness could be seen as something that can be broken down. Often we perceive consciousness as a gestalt, or a whole. It’s a binary, on or off switch. You got it, or you don’t.
PGS discusses one primary theory for how consciousness works, which comes from someone who it didn’t work for - at least, not quite in the same way:
One path begins with an accident, a case of carbon monoxide poisoning from a shower’s bad water heater in 1988, which led to a case of brain damage in a woman known only as “DF”. As a result of the accident, DF felt almost blind. She lost all experience of the shapes and layout of objects in her visual field. Only vague patches of colour remained. Despite this, it turned out that she could still act quite effectively toward the objects in space around her. For example, she could post letters through a slot that was place at various different angles. But she could not describe the angle of the slot, or indicate it by pointing. As far as subjective experience goes, she couldn’t see the slot at all, but the letter reliably went in.
As PGS explains, after studying DF for some time, David Milner and Melvyn Goodale proposed that visual information moved through the brain in two streams; the ventral stream, which was concerned with categorisation and description, and the dorsal stream, which was concerned with real-time navigation through space. The dorsal stream would have allowed DF to place things through the letter-box, but the ventral stream may provide conscious experience of doing so. In this account, DF had lost her ventral stream, and so had no conscious experience, but could continue to complete tasks.
In the 1960s, David Ingles rewired frog nervous systems, swapping the wires in the brain. Frog nervous systems have turbo-regeneration powers, so this was less difficult than it sounds. Ingles produced a frog that snapped at prey to its left when the prey was to the right, and vice versa. Swapping vision did not create a complete metamorphosis however, and when the frog was presented with barriers, it just moved around them in a normal way.
So only certain things were swapped, kinda like how Freaky Friday conveniently ignored the issue of embodiment - if Jamie Lee Curtis’ mind really was in Lindsey Lohan’s body, she would have struggled to walk around for, in my estimation, at least a day, while she relearned spatial mappings for a smaller body. This is why I had to give it only 95% on RottenTomatoes.
Milner and Goodale:
So what did these rewired frogs “see”? There is no sensible answer to this. The question only makes sense if you believe that the brain has a single visual representation of the outside world that governs all of an animal’s behaviour. Ingle’s experiements reveal that this cannot possibly be true.
The experience of consciousness in this account emerges from some combination of those two streams, the dorsal and the ventral, which together generate the internal model that we use to represent the external world.
According to Stanislas Dehaene, consciousness seems to be critically important for learning things over periods of time, for things that come in sequences, and for things that are new. You can use and do use consciousness for other things, but those are his keystones of the consciousness arch.
For instance, you can flash a sequence of words at someone, so fast that they will tell you that they didn’t see anything. But if you measure their brain while this happens, it responds differently to sentences which have incongrous meanings - say, “joyful dying grandma” - to ones that have meanings that make more sense - say, “sad constipated grandma”.
If a light is shone at someone and immediately followed by an irritating puff of air, people learn to blink. If there’s a second gap between the light and the puff, people don’t learn to blink without conscious attention. So there are multiple sets of processes going on, some of which tap into consciousness, and some of which sit below the surface. You only get to see that top layer of consciousness, bound together and neatly unified.
This idea that consciousness is singular is known as the unity thesis, and you could also express this as the idea that you can only have one set of subjective experiences at any one time. Another important idea when considering consciousness is the idea that it is neurally instantiated, i.e., that it arises from nervous systems. Most consciousness theorists are physicalists, and so consequently believe that consciousness should be shaped by the structure of the nervous system. This is known as the isomorphism thesis.
The important idea that emerges from all of this comes from Tim Bayne, who writes that “some creatures simply won’t have the cognitive machinery required to integrate the contents of mental states in an appropriate manner”.
There are some humans whose corpus callosum - a big white matter tunnel that connects the two hemispheres of the brain - has been severed, usually to lessen the severity of epileptic attacks. Split-brain patients can have two experiences of consciousness, and an important adjunct is that those experiences of consciousness can exist in such a way that neither is aware of the other.
If a compound word like “key-ring” is presented to split-brain patients, such that the right hemisphere can only see “key”, and the left hemisphere can only see “ring”, the patient verbally reports a “ring”, because language is in the left hemisphere. However, the patient can grasp a “key” with her left-hand (remember, the right hemisphere controls the left half of the body), even if they can’t issue verbal reports about what they’re doing.
As I mentioned above, octopuses don’t have somatotopic representations of their arms in their brain, and its thought that neural circuitry in the arms isn’t complex enough to generate anything more than primary consciousness or “direct awareness of the world without further reflection upon that awareness”.
So the arms may have primary consciousness, while the brain may have some form of higher-order consciousness, which allows for reflection. These reflective processes come online in response to complex situations, such as when the octopus brain thinks, “there’s some food through this bizarre labyrinth the flesh walkers have constructed, let me help my arms get to it.” Although, obviously, its thoughts would probably be nothing like that.
So what might it feel like in the octopus brain, if they don’t have the neural machinery to bind their different conscious fields? What does it feel like to have a non-singular consciousness?
PGS argues for the idea I discussed above - that octopuses have some sort of global command centre operating in the brain, but that their control of any specific situation is down to local fine-tuning in the arms. When the octopus brain and arms start operating together, the brain probably doesn’t gain conscious experience of the arms.
This seems likely, because humans who have problems with their proprioception often say that this is how their conscious experience works. For instance, Ian Waterman, who lost all proprioceptive sensation from the neck down, operated mainly by visually guiding his body.
PGS discusses another example in Other Minds, where even people without impairment can lack awareness of proprioceptive action:
Bence Nanay, a philosopher based in Antwerp, also sent me a quite different interpretation of the octopus/human comparison. Bence thinks that some relationships that look weird and novel in the octopus case are present in our case too, if we look hard enough. They are usually invisible to us, but they are there. Suppose you are reaching for an object with your hand. If the location or size of the target you are reaching for suddenly changes, your reaching movement changes extremely quickly - in less than a tenth of a second. This is so fast that it is unconscious. Subjects in experiments don’t notice the change - they don’t notice that they’ve changed their own movement, and don’t notice the change in the target object. When I say “subjects” in the experiment, I mean that if you ask the person if there was a change, they will say no. The person does not notice the change, but their arm alters its path.
As in the octopus, there’s a top down decision to extend the hand, but also a fine-tuning which is fast and unconscious. In the octopus case, the fine-tuning is greater - it’s more than just fine-tuning - and it does not only happen quickly. The octopus might watch some of the arm’s wandering as if it is just a spectator.
As Chiel and Beer put it in this paper:
The nervous system is one of a group of players engaged in jazz improvization, and the final result emerges from the continued give and take between them. In other words, adaptive behavior is the result of the continuous interaction between the nervous system, the body and the environment, each of which have rich, complicated, highly structured dynamics. The role of the nervous system is not so much to direct or to program behavior as to shape it and evoke the appropriate patterns of dynamics from the entire coupled system. As a consequence, one cannot assign credit for adaptive behavior to any one piece of this coupled system.
They’re referring to humans, but PGS is right that the metaphor is perhaps more apt for octopuses, whose cognition is clearly more distributed. Another musical metaphor is offered by O’Brien and Opie, who suggest a form of multi-track polyphony might represent consciousness, and Carls-Diamante moots this as a possibility for octopus consciousness. As she puts it:
[O’Brien and Opie] introduce a multi-track polyphonic model that construes the field of phenomenal experience as an amalgam of distinct cross-modal contents experienced simultaneously. Based on an analogy to music, the polyphonic model holds that multiple conscious contents are experienced simultaneously, i.e. as part of a single experience. Polyphony is correctly understood as the mingling of voices to produce a single sound wave, in which case a single experience arises from the contribution of distinct components. While the end result is a coherent and connected product, the components do not lose their independent qualitative properties, so it is still possible to identify and distinguish between the parts.
I like this idea of multitudes within one, of discrete parts being pulled in and out of the fray of the mind in order to generate wholes that quickly fragment and atomise as the problem changes and the currents shift. I also like the notion that we could somehow Fourier transform a person’s brain waves into the distinct parts of consciousness that constructed those waves. It seems coherent, in keeping with what we know about the nervous systems of cephalopods, and also, to my eyes, a beautiful theory. Sadly, we never do get to really find out what it is like to be something else. We just have to keep guessing, in slowly more informed ways.
Other Minds was written five years ago now, and the science on the octopus is slowly getting richer with every passing year. In writing this piece I am indebted in particular to three papers; Jennifer Mather’s What Is In An Octopus’ Mind?, Sidney Carls-Diamante’s The Octopus and the Unity of Consciousness, and Michael Trestman’s The Cambrian Explosion and the Origins of Embodied Cognition.
Nonetheless, octopus science still moves relatively slowly, and much of the work that PGS examines doesn’t seem to have been superseded. We probably shouldn’t expect rapid developments anyway - the fact that they are aquatic, temperamental creatures is not conducive to quick breakthroughs. But they do offer a fascinating counter-example to much of the discussion about consciousness and cognitive capabilities, a squishy blob of mollusc that is mischievous, playful and capable of firing ink at your face.
They are, as PGS is fond of saying, “independent experiments in the evolution of the mind”. And for that reason, they’re worth spending some time with, even if only because, if they could survive on land, they seem like they’d be a blast at parties.
I am sympathetic to most people who fall afoul of crypto scams, but some people did invest a combined $300,000 into Monkey Jizz ($MJIZZ), so I’m less sympathetic to those people.